Visit UCR Return to Campus website - Take the COVID Screening Check survey
Colloquium: Dr. Kyle D. Squires
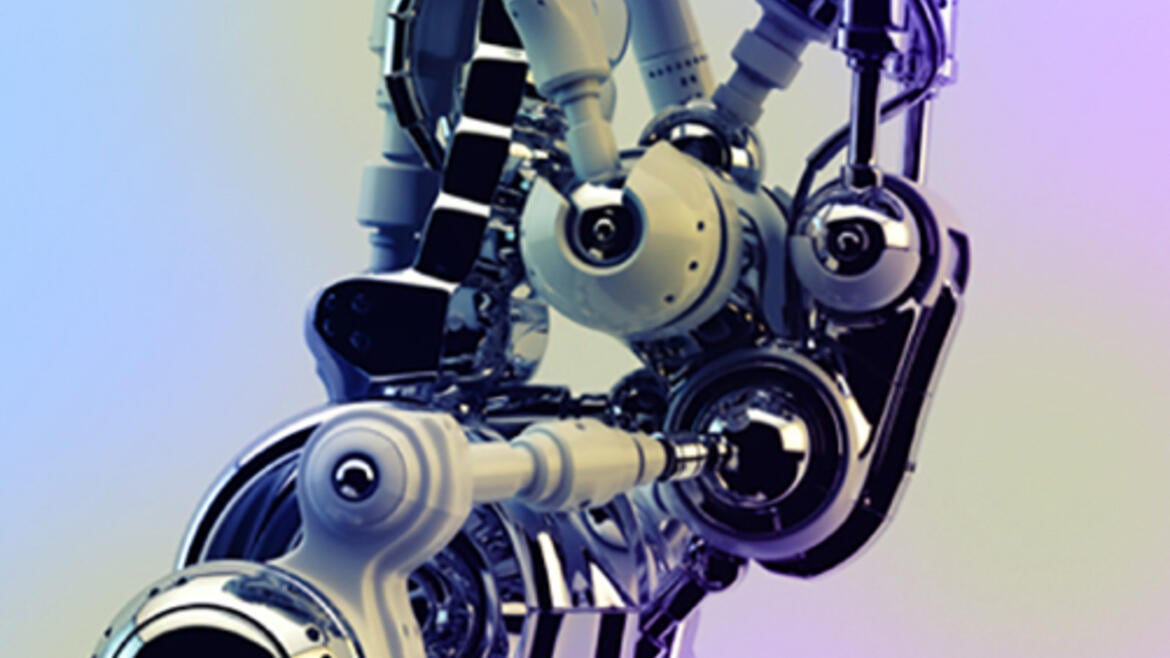
Direct Numerical Simulation of the Flow around a Golf Ball
Distinguished Speaker
Dr. Kyle D. Squires
Mechanical and Aerospace Engineering Department
Arizona State University
Tempe, Arizona
Since the accidental discovery of drag reduction by surface roughening, golfers and equipment manufacturers have sought to optimize dimple patterns to reduce drag. Optimisation of dimple patterns - their density, shape, and size - requires an understanding of the mechanisms that lead to drag reduction. While it is well known that dimples induce the drag crisis at lower Reynolds numbers than for a smooth sphere, the controlling mechanisms are not well understood. The goal of the present study is the application of Direct Numerical Simulation (DNS) to the flow around a golf bal in order to understand the role of surface dimpling. The numerical approach is based on an immersed boundary technique in which a distribution of forces is used to represent the effect of the golf ball surface on the flow. DNS results are reported for simulations at two Reynolds numbers, pre- and post-drag crisis. The computations are performed using as many as 1.2 billion grid points.
Flow visualizations reveal the delay of complete flow detachment due to local separation and reattachment within individual dimples. Mean velocity profiles indicate the local separations evolve into complete detachment of the flow in the subcritical case around 84 degrees (measured from the front stagnation point), while in the supercritical regime it leads to alternating regions of reattachment and separation until the flow detaches completely around 110 degrees. Predictions of the drag coefficient are in reasonable agreement with measurements. Energy spectra highlight frequencies associated with vortex formation in the shear layer of the supercritical regime. Statistics of the Reynolds stress quantify momentum transport in the near-wall region by displaying trends of the radial and axial principal stresses. These stresses increase in magnitude around 84 degrees for the subcritical case, while in the supercritical case these stress components alternatively increase and decrease in magnitude, corresponding to local separation and detachment.
Dr. Kyle D. Squires currently serves as Chair and Professor of the Department of Mechanical and Aerospace Engineering in the Ira A. Fulton School of Engineering. Dr. Squires earned his Ph.D. in Mechanical Engineering from Stanford University in 1990; he was a post-doctoral research associate at the Center for Turbulence Research from 1990-1991 where he worked on subgrid-scale modeling of compressible turbulence. His first faculty appointment was in the Mechanical Engineering Department at the University of Vermont beginning in 1991. He joined the faculty at Arizona State University in 1997. Professor Squires' research expertise encompasses computational fluid dynamics, turbulence modeling of both single-phase and multi-phase flows, and high-performance computing. Specific interests include the use of direct numerical simulation and large eddy simulation applied to particle-laden turbulent flows and the development of hybrid Reynolds-averaged and large eddy simulation techniques for high Reynolds number wall-bounded flows. Professor Squires applies his expertise exploring ways to improve the aerodynamics of aircraft, ground vehicles, and sports equipment. Dr. Squires has held numerous visiting appointments in the US, Japan, and Europe and was elected a Fellow of the American Physical Society in 2008.